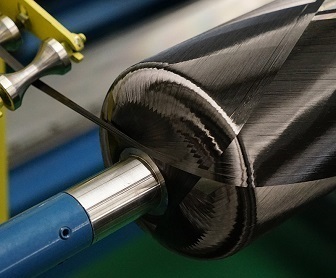
Composite Materials and Preparation Process for Type IV Composite Hydrogen Storage Cylinder
Hydrogen is a flammable and explosive gas. High pressure gaseous hydrogen storage has fast charging and discharging speed, and can be operated at room temperature. However, high-strength pressure resistant containers are required. The diameter of hydrogen atoms is only 0.982nm, which may penetrate or cause metal deterioration in metal materials, resulting in hydrogen embrittlement, corrosion of pressure vessels, and risks of leakage and explosion, especially under high pressure. In 2002, the U.S. Department of Energy divided hydrogen storage cylinders into four categories:
At present, the most mature hydrogen cylinder for fuel cell vehicles in China is the Type III cylinder, which is produced by manufacturers such as Guofu Hydrogen Energy, Sinoma Technology, and Kotec; The IV bottle technology is not yet mature and cannot be mass-produced, and is basically monopolized by foreign manufacturers. For example, the world's largest IV bottle manufacturer, Hexagon Hexconn, as well as Toyota Gosei and Iljin's IV bottles, have been used in Toyota Mirai series fuel cell vehicles and Hyundai Nexo series fuel cell vehicles, respectively. The manufacturing cost of Type IV hydrogen storage bottles ranges from 3000 to 3500 US dollars, mainly including composite materials, valves, regulators, assembly inspections, hydrogen, etc. The cost of composite materials accounts for over 75% of the total cost, while the cost of hydrogen itself only accounts for about 0.5%. The development trend of hydrogen storage bottle technology is lightweight, high pressure, high hydrogen storage density, and long service life. Compared to traditional metal materials, polymer composite materials can reduce tank wall thickness, improve capacity and hydrogen storage efficiency, and reduce energy consumption costs during long-distance transportation while maintaining the same pressure resistance level. Therefore, the performance and cost of composite materials are key to the preparation of type IV hydrogen storage cylinders.
Structure of Type IV Hydrogen Storage Cylinder
The cylinder body of the IV type hydrogen storage cylinder, except for the metal valve seat, is all made of non-metallic composite materials.
Due to the fact that the IV type cylinder body is all resin and easy to shape, its external dimensions can be adjusted according to the design requirements of different manufacturers and models of fuel vehicles.
Cylinder forming process
First, the thermoplastic olefin polymer is made into an inner liner, and then the surface is checked for defects such as wrinkles and dents. Then, the fiber winding process is carried out, and the label is affixed before curing. Then, the outer surface is polished, and after passing the static pressure test, the final finished product inspection is carried out.
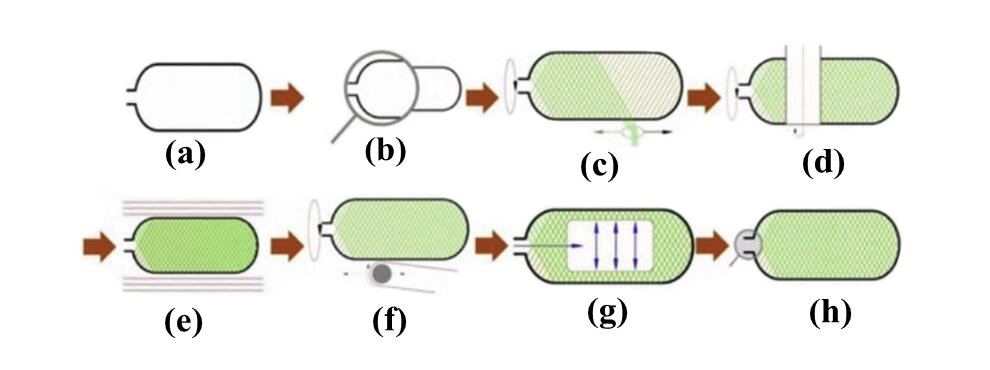
The corresponding process for letters is (a) non-metallic lining, (b) lining inspection, (c) fiber winding, (d) adhesive labeling, (e) curing, (f) surface polishing, (g) static pressure testing, and (h) finished product inspection. The key processes are detailed below.
1. The first process of manufacturing a type IV hydrogen storage bottle with a rotational plastic inner liner is to prepare an inner liner with hydrogen barrier properties. The process is shown in Figure 3.
The corresponding process for letters is (a) mold filling, (b) dual axis rotation, (c) cooling, and (d) product demolding
Put polyolefin powder with high melting index and good fluidity into a rotational molding mold. The mold is heated above the melting point of polyolefin, and two different axes rotate the mold simultaneously. After the powder is melted, it is evenly distributed into a hollow structure inside the mold. After forming, it is cooled and demolded, forming a roughly cylindrical bottle with a uniform radius. The advantages of rotational molding process are uniform wall thickness and simple molding process, but there is also the problem of low product density and easy formation of defects, so the inner liner wall thickness cannot be too thin. When the formed inner liner enters the next process and is wrapped with carbon fibers, it will be concave inward under pressure, so a certain amount of air pressure should be filled inside to balance the tension of the fibers.
1. The middle and outermost layers of the filament wound hydrogen storage bottle are both made by the winding process. Fiber winding is one of the most advanced methods for manufacturing hollow composite components, which can produce tanks of 1-100m3. The carbon fiber reinforced resin winding process is shown in Figure 4, including winding unit, resin impregnation unit, and control unit.
Among them, W is resin impregnated carbon fiber, CF is carbon fiber, EP is epoxy resin, and Ip is inorganic particle. Carbon fiber dry wire is wound on multiple roving winding frames, guided by fixed pulleys, fully immersed in liquid epoxy resin, and then assembled into a 1cm wide ribbon fiber bundle. Under precise computer control, the fiber bundle is repeatedly wound around the outer periphery of the inner liner through the axial reciprocating motion of the laying box, combined with the rotating driving device of the inner liner, to obtain the intermediate product tank. The liquid tank equipped with epoxy resin is equipped with a mechanical stirring device to ensure that inorganic ferrite particles with microwave absorption can be evenly dispersed, thereby improving thermal curing performance, and constantly replenishing fresh liquid epoxy resin. The molecular chains of the fibers are oriented along the direction of force while being stretched, and appropriate stress is pre applied to the fibers through additional equipment before winding, which can make the winding position of the fibers on the inner liner more precise. In addition to the inherent strength of composite materials, the molding method is also crucial for its performance impact. There are infinite possibilities for the combination of types of winding (end winding, spiral winding, and hoop winding), frequency, direction, width of winding tape, spacing, etc., and it is necessary to rely on CAE computer-aided engineering design.
2. The focus of the safety performance evaluation of the microwave cured IV type hydrogen storage bottle body is the mechanical properties of carbon fiber and glass fiber reinforced epoxy resin composite materials, including matrix cracking, fiber/matrix debonding, and fiber fracture. Fiber reinforced epoxy resin usually takes several hours to cure, and the thermal diffusion coefficient between the fiber and the resin matrix is different, making the interface more susceptible to stress concentration or relaxation, reducing the performance of the composite material. Under microwave irradiation conditions, the curing time can be significantly shortened, reducing the residual internal stress of the cured material, increasing the interfacial force between the substrate and fibers, thereby improving the strength and stiffness of the composite material. The microwave irradiation curing process is shown in Figure 4.
The tank shafts at both ends of the tank support the intermediate product tank on the frame, rotating at a constant speed. A 2.1GHz microwave is generated through a microwave oscillator, which directly irradiates the fiber reinforced resin layer of the intermediate product tank and reflects on the inner wall surface of the microwave heating device. Then, it continues to be applied to the fiber reinforced resin layer, where the epoxy resin is cured by microwave heating, and then cooled to obtain a high-pressure hydrogen tank. The duration of microwave irradiation is determined by the size of the final high-pressure hydrogen tank product, the thickness of the fiber reinforced resin layer, and the number of times the fibers are wound. In order to improve strength, the above steps of fiber winding and microwave curing can be repeated multiple times. The ratio of composite materials such as carbon fiber, glass fiber, and epoxy can be adjusted as needed, and the post curing process can be added, that is, the epoxy resin can be fully cured by placing it at an appropriate high temperature for a long time. Due to the longer winding time, the resin's wettability will deteriorate, and the higher system viscosity will make it difficult to expel bubbles. Therefore, it is necessary to add low molecular weight and low viscosity epoxy resin for blending, and the viscosity of the outermost layer of epoxy resin should be lower than that of the middle layer of epoxy resin to facilitate the discharge of internal gases during the curing process.
1. Pre impregnation process fiber winding process includes: wet winding, dry winding, and semi dry winding
Figure 5 shows the process diagram of dry fiber pre impregnation. The dry fiber is pulled by a roller and fully impregnated in a liquid epoxy resin tank. After that, it is preliminarily formed through multiple independent small mold holes arranged closely on the mold. It is then semi cured and shaped by heating in the final mold, and then cut into pre impregnated cloth.
Due to the extremely narrow guide channel, the frictional effect of fibers during passage can generate fuzz, dry fibers, dirt, and excess surface resin, resulting in blocked guide channels and reduced productivity. Dry fibers and pre impregnated cured fibers can be weighed separately, thus accurately measuring the amount of epoxy resin contained in each fiber. After the preparation of pre impregnated fibers, attention should be paid to their storage conditions. When needed, the pre impregnated cloth should be heated to above 140 ℃ before being wound onto the inner liner. The curing conditions such as the amount of epoxy resin contained and the required temperature and time can be better controlled.
Composite
1. The hydrogen barrier material used in hydrogen storage bottles is the key to ensuring the safety of hydrogen barrier. Figure 7 compares the gas permeability of different polymer materials, and the barrier properties of EVOH ethylene vinyl alcohol copolymer for oxygen, water vapor, and carbon dioxide are far superior to other polymer materials.
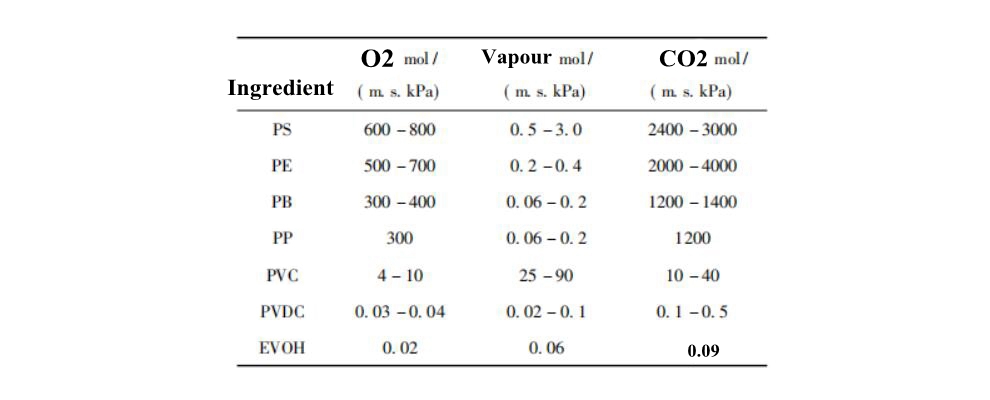
Although PVDC polyvinylidene chloride has excellent barrier performance, it is not suitable for gas storage cylinder liners that come into direct contact with hydrogen gas due to the residual trace amount of chlorine gas reacting with hydrogen gas. EVOH is a copolymer of ethylene and vinyl alcohol, with a general ethylene content ranging from 20 to 45mol%, a density of 1.13 to 1.31g/cm3, and a melting point of 160 to 190 ℃. EVOH combines the gas barrier properties of PVA polyvinyl alcohol and the processability of PE polyethylene. By adding EVOH, the inner liner of the hydrogen storage bottle remains elastic even at low temperatures, ensuring excellent barrier performance. The high gas barrier of EVOH is mainly due to its high crystallinity and hydrogen bonding of hydroxyl groups. When the ethylene content decreases, the gas barrier increases; When the ethylene content is greater than 50mol%, the gas barrier will be severely damaged. The gas resistance decreases with increasing temperature, such as when the temperature increases from 20 ℃ to 35 ℃, the oxygen transmittance increases by more than three times. When the relative humidity is greater than 30%, the interaction between water molecules and hydroxyl groups in EVOH will lead to a significant decrease in gas barrier performance. The permeation of hydrogen gas can lead to two problems: (1) plastic inner liner instability and inward collapse. (2) The plastic inner liner material undergoes yielding and even blistering and cracking. Special attention should be paid to the structure and material sealing between the metal filling port and the polymer bottle body for type IV hydrogen storage cylinders. Graft polymers can be used to modify the inner liner material, such as adding only 1wt% low viscosity EVA g-MA, which can significantly improve the viscosity and torque of EVOH. In order to ensure long-term safe use of EVOH in IV type hydrogen storage cylinders at 70MPa, the following issues need to be addressed: (1) Residual hydrogen in the resin causes resin foaming. (2) Hydrogen embrittlement causes claw shaped cracking on the surface of the resin. (3) Durability to withstand repeated stress loads at a low temperature of -40 ℃. (4) Maintain gas resistance under high pressure conditions. At present, there are only three companies worldwide that produce EVOH resin. Since the first development of the synthesis process for EVOH in 1972, Japan's Corolla Corporation has been the world's largest production enterprise, with its brand name being EVAL ®, The other two are Japan Synthetic Chemical Industry Corporation and Taiwan Changchun Petrochemical. China's annual consumption of 20000 tons of EVOH is largely dependent on imports.
1. Carbon fiber reinforced material hydrogen gas is compressed to 70Mpa and stored in the storage tank. It is necessary to ensure that the strength of the main body can withstand high pressure. When the hydrogen gas in the storage tank is consumed, the main body also contracts with the decrease of pressure. High pressure environment and repeated inflation and deflation can lead to material fatigue. The carbon fiber used for hydrogen storage bottles mainly consists of Dongli high-strength carbon fiber filament TORAYCA ® T1000G, T1100G products. T1000G and T1100G are made of polyacrylonitrile as raw materials, using nanoscale fine control fiber structure firing technology to achieve high strength and high elastic modulus, and nano alloys of composite materials are prepared through pre impregnation process. The high-strength carbon fiber used as a storage tank material not only has impermeability, but also excellent heat and chemical resistance, as well as good dimensional stability, strength, and wear resistance. Carbon fiber bundles with an average diameter of 5-8 μ Composed of 10000 to 50000 carbon fibers. The weight ratio of epoxy resin layer to carbon fiber layer is 20-30:70-80. There is not only physical adhesion but also chemical bond force between the skeleton carbon material and the adhesive. Aromatic epoxy resin provides higher strength than aliphatic epoxy resin such as bisphenol A epoxy resin. However, high viscosity can make the impregnation operation difficult and difficult to evenly impregnate the interior. Therefore, it is necessary to use epoxy resin or solvent with low polymerization degree to adjust the viscosity during impregnation. The disadvantage is that repeated impregnation is necessary to regulate large fluctuations in volume shrinkage rate. Although domestic T800 and T1000 high-performance carbon fibers have successfully broken through laboratory related preparation technologies, achieving industrial transformation still requires key development and improvement in supporting technologies such as raw materials, equipment, and process control. Especially not only does it involve the preparation of high-strength carbon fiber dry fibers, but also the pre impregnation of high-strength carbon fibers, which completes the weaving process. Dongli is the world's largest producer of carbon fiber, and has formed an industrial cluster covering the entire carbon fiber industry chain with Japanese companies such as Emperor and Mitsubishi.
2. The viscosity of epoxy resin used for hydrogen storage tanks is 4000-8000cP at 25 ℃, and the solid particle size is less than 10-25 μ m。 The glass fiber in the outermost fiber reinforced resin layer has low thermal conductivity, so it should be ensured that the equivalence ratio of epoxy resin to curing agent is 1:1, and the inorganic ferrite particles that can absorb microwaves should be evenly dispersed to achieve sufficient curing, or the fiber should be strengthened by heating after irradiation with microwaves. During the heat curing process of epoxy, the polyolefin liner will soften. In order to protect the liner structure, the maximum curing temperature should be controlled below the liner softening point by more than 10 ℃. Under high pressure, hydrogen gas penetrates into the material and gradually diffuses to the interface of the composite material, while the repeated charging and unloading of hydrogen gas forms a pressure difference, ultimately leading to yielding, bubbling, and interfacial tearing of the inner liner material. The improved method is to add clay to the composite material.
As shown in Figure 7, carbon fiber is impregnated with an epoxy resin matrix, and then laminated with two layers of clay films with a thickness of 1nm, respectively, under high temperature and pressure conditions to plasticize and heat form them. A semi cured prepreg is prepared in the form of flakes, and finally subjected to post curing treatment at 100-150 ℃ for 10-30 hours. Carbon fiber is used as the skeleton material, and epoxy resin is used as the adhesive. The clay film has the effect of enhancing adhesion and sealing, mainly composed of clay, containing a small amount of resin, and has a uniform thickness without pinholes. Even after 100 exposures at -196 ℃, the hydrogen barrier performance of this composite material remains almost unchanged. The localization and popularization of the entire hydrogen energy industry chain require the underlying support of material science. Only by systematically connecting relevant research and industrialization work, and establishing a complete database system from macro process parameters, to microstructure indicators, and then to macro usage performance, can it be possible to rise from a single dimensional perspective to a multi-dimensional perspective, and then discover and apply higher-level laws. When our understanding and operation level of material control accuracy are improved, we will naturally be able to adjust the product structure and supply according to demand, realize the optimization, low-carbon and sustainable energy structure in China, and finally achieve the ultimate goal of energy independence and carbon neutrality.